Dna Manipulating Enzymes Pdf
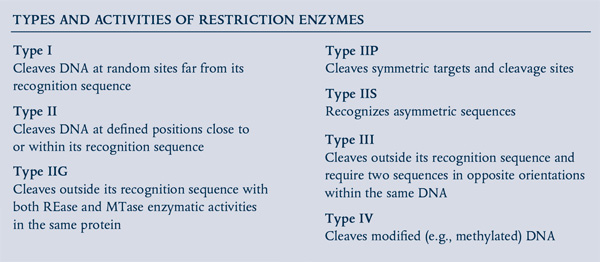
Abstract Mechanical manipulation of single DNA molecules can provide novel information about DNA properties and protein–DNA interactions. Here we describe and characterize a useful method for manipulating desired DNA sequences from any organism with optical tweezers. Molecules are produced from either genomic or cloned DNA by PCR using labeled primers and are tethered between two optically trapped microspheres. Software Maya 5.0.
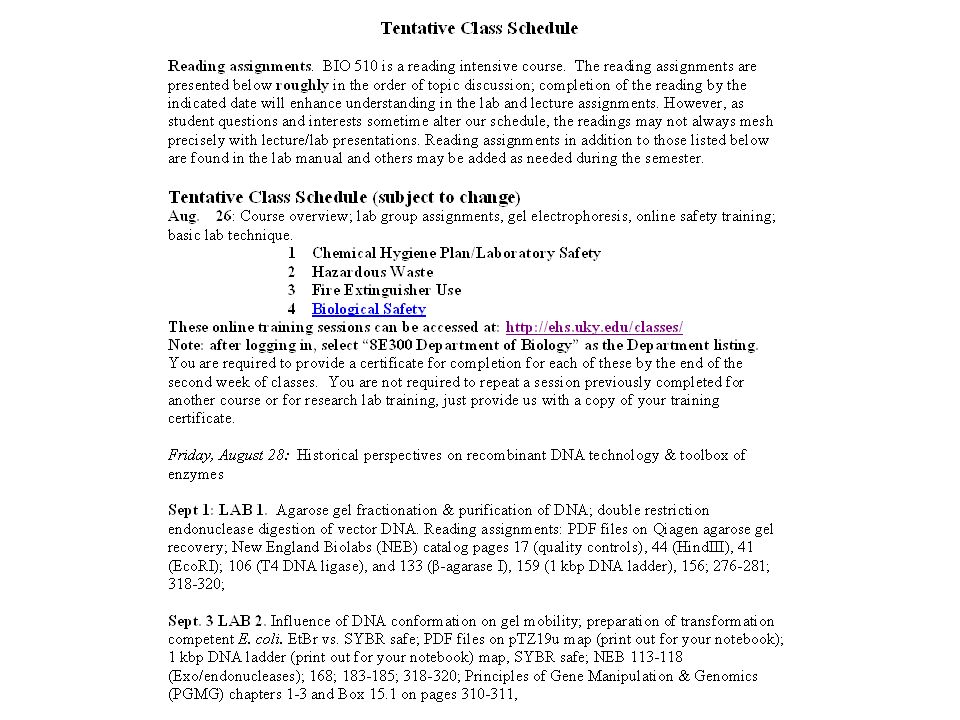
We demonstrate that human, insect, plant, bacterial and viral sequences ranging from ∼10 to 40 kilobasepairs can be manipulated. Force-extension measurements show that these constructs exhibit uniform elastic properties in accord with the expected contour lengths for the targeted sequences. Detailed protocols for preparing and manipulating these molecules are presented, and tethering efficiency is characterized as a function of DNA concentration, ionic strength and pH. Attachment strength is characterized by measuring the unbinding time as a function of applied force.
An alternative stronger attachment method using an amino–carboxyl linkage, which allows for reliable DNA overstretching, is also described. INTRODUCTION Protein–DNA interactions play a critical role in the molecular biology of all organisms.
For example, the ∼3.3 billion base pairs human genome is estimated to code for at least several thousand DNA-binding proteins, including transcription factors, nucleases, repair proteins, topoisomerases, structural proteins, and DNA and RNA polymerases. A wide variety of methods exist for studying protein–DNA interactions, including DNase footprinting, sucrose gradient sedimentation, gel mobility shifts, fluorescence spectroscopy, imaging by electron microscopy and X-ray crystallography. Over the last decade another approach involving mechanical manipulation of single DNA molecules has been developed. Manipulation of DNA by optical tweezers was pioneered by Chu and co-workers, and extended by Bustamante and co-workers ( – ). This method has since been applied to study many fundamental biochemical processes, including transcription, replication, chromatin unraveling, viral DNA packaging and helicase translocation ( – ).
Because protein–DNA interactions are vital to all organisms and these interactions are often sequence dependent, it is desirable to have a general method for manipulating DNA sequences from any organism. Here we describe and characterize such a general method in which selected DNA sequences from a variety of organisms are tethered between two microspheres. Besides providing useful protocols and a characterization designed to optimize the efficiency of the method, this work also serves to test the notion that the global elastic properties of these long, AT-GC balanced DNA molecules are largely independent of sequence (as opposed to the local properties on a = 5040 nm with a SD of σ = 29 nm, while for the construct produced from the BAC clone (measured ∼1 month later) we obtained = 5080, σ = 47 nm. This correspondence suggests that the two constructs are the same, at least to within tens of nanometers, and is consistent with our estimate of ∼10 nm/week long-term instrumental drift. Next we sought to check that the molecules were behaving in a manner consistent with the targeted construct lengths. The elasticity of DNA molecules has been shown to agree with the behavior predicted by the worm-like chain (WLC) model (,, – ). In this model, the fractional extension of a molecule (defined as the end-to-end distance divided by the contour length) is a universal function of the applied force.
The extension of molecules of different contour lengths at a given force is therefore expected to be proportional to the contour length ( ). To confirm that this was true for all of our DNA constructs we compared the ratios of average molecular extensions measured at 25 pN to the ratios of number of basepairs of the constructs. We chose to calculate ratios instead of absolute values because such ratios should depend only on the relative lengths of the constructs and should therefore be independent of instrument calibration. As shown in, there is excellent agreement between the measured and predicted ratios.
The average deviation from the expected ratio is only 0.3% and the largest deviation is only 2%. Furthermore, when force is plotted versus fractional extension, all of the datasets collapse quite well onto a single curve, as predicted by the WLC model ( ). These results confirm that our method of preparing and tethering DNA sequences yields optical tweezers measurements that are highly repeatable and consistent with the targeted sequences. It is known that on short length scales (∼10–100 bp) different DNA sequences may have dramatically different conformations and bending and torsional rigidities (, ).